Home / Insights / Whitepaper /
Analysis on the Impact of Laser Marking on the Fatigue Life of Metal Orthopedic Implants
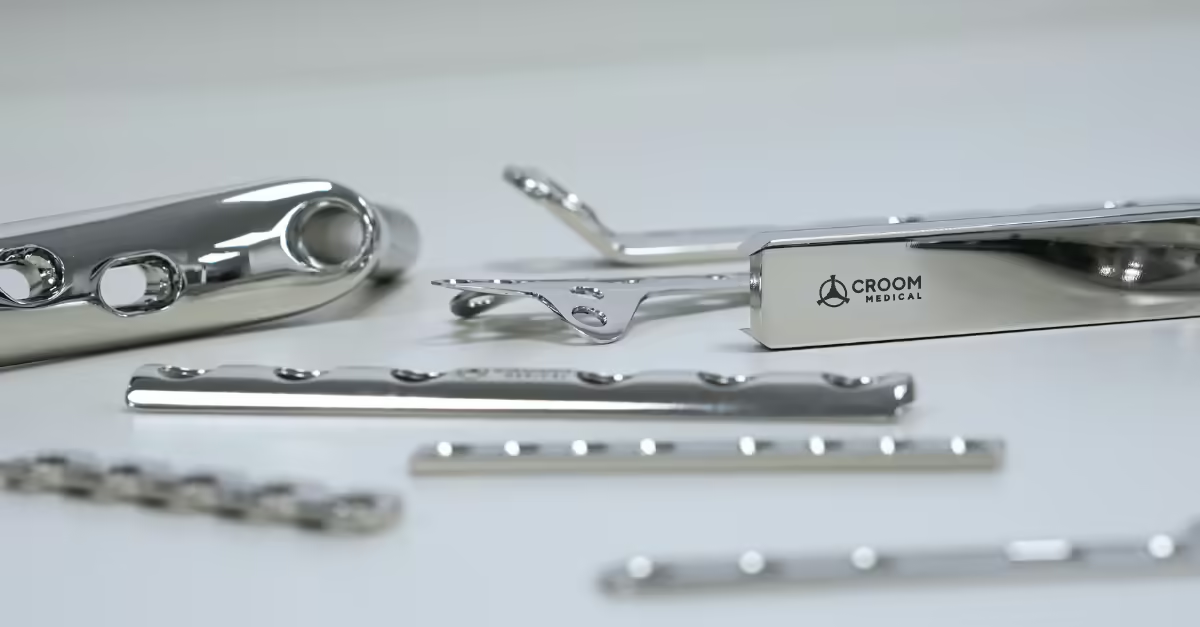
Introduction
Laser marking is a key process in orthopedic implant manufacturing, playing an essential role in product identification, traceability, and regulatory compliance. Implants carry critical information such as lot numbers, manufacturer details, and size specifications to:
1. Ensure the correct implant is selected during surgery.
2. Facilitate efficient product recalls, when required.
3. Enable long-term tracking of implant performance.
In the highly regulated medical device industry, traceability is not just best practice—it’s a legal requirement. The FDA’s Unique Device Identification (UDI) system requires that medical devices, including orthopedic implants, carry a unique identifier for compliance (FDA, 2023). At Croom Medical, integrating these requirements ensures that every implant has a durable and legible identifier, supporting both traceability and surgical success.
What is fatigue in metal implants?
Fatigue in metal orthopedic implants refers to the progressive structural damage that occurs under repeated loading, such as the forces from walking or climbing stairs. Fatigue life—the duration an implant maintains structural integrity—is crucial to its success. Factors influencing fatigue life include:
- – Material properties
- – Surface finish
- – Residual stresses
- – Presence of stress concentrators
Understanding how laser marking impacts these factors is vital to ensure implant longevity and patient safety.
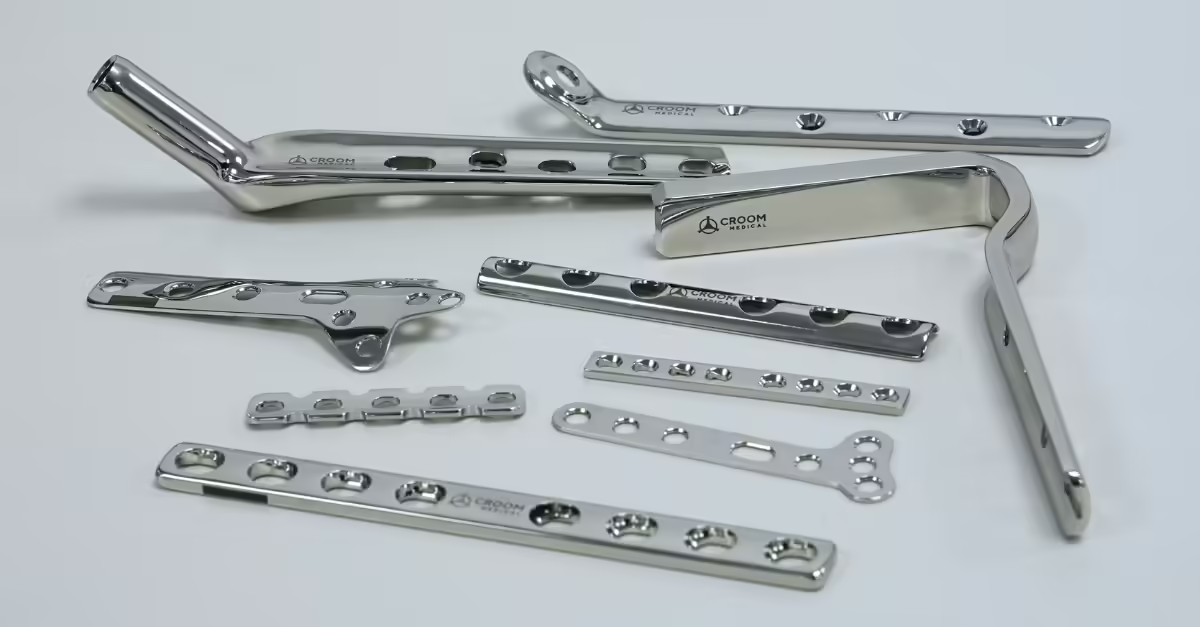
Figure 1 – Laser marking on bone plates manufactured by Croom Medical. The laser marking process is optimised to ensure durability and compliance with traceability standards while minimising any potential impact on the implant’s fatigue life.
Laser Marking Technologies for Orthopedic Implants
Laser marking is often preferred for implant identification because of its precision, versatility, and the permanent, contact-free markings it produces. At Croom Medical, our laser marking methods include:
- – Laser Engraving: Uses vaporisation to create deep, high-contrast marks.
- – Laser Etching: Melts the surface for shallow, raised or depressed markings.
- – Laser Annealing: Uses controlled heating to create oxide layers, resulting in color changes without material removal.
To minimise adverse effects on implant integrity we use advanced fiber and ultrafast laser systems. Each type of laser offers unique advantages, such as CO2 lasers for non-metals, fiber lasers for efficient metal marking, and ultrafast lasers to limit heat-affected zones. Croom Medical utilises Trumpf TruMark Station 5000 and HK Laser systems in its operations.
Figure 2 – Laser Annealing on an Orthopaedic Implant. The laser beam heats the implant surface to a controlled temperature, creating an oxide layer that imparts a distinct colour. This process allows for durable, non-invasive marking without removing material, preserving the implant’s surface integrity. Source: TRUMPF
Figure 3 – Laser Engraving on an Orthopaedic Implant. The laser beam removes material from the implant surface, creating a precise, recessed marking or engraving. This technique ensures clear and durable identification while maintaining the structural integrity of the workpiece. Source: TRUMPF
Impact of Laser Marking on Fatigue Life
Scientific Literature and Studies
Research indicates that laser marking can significantly influence the fatigue strength of metal implants due to surface alterations during marking. For example, Nikolaj et al. (2023) found that laser marking can reduce the fatigue strength of titanium alloys, commonly used in implants, due to surface features acting as stress risers. At Croom Medical, we have collaborated with leading academic institutions like UL, QUB, UCD, and TCD to study these effects.
Analysis of Material Property Changes
The thermal processes of laser marking can lead to:
- Microstructural Changes: Rapid heating and cooling can alter the microstructure, sometimes creating brittle phases that compromise fatigue life (Lucideon, 2023).
- Residual Stresses: Induced by the thermal cycle, these stresses can be beneficial (compressive) or detrimental (tensile) to fatigue resistance (Henriksen et al., 2023).
- Surface Roughness: Increased surface roughness can act as a stress concentrator, potentially initiating fatigue cracks (Pompe et al., 2003).
These impacts vary based on factors such as laser power, pulse duration, and implant material characteristics. At Croom Medical, our R&D initiatives provide insights into these parameters, enabling us to refine our marking processes to reduce adverse effects.
Figure 4 – Cross-sections of laser-marked CP-Ti specimens showing cracks penetrating beyond the oxide layer, varying with laser parameters.
Case Studies and Experimental Data
In collaboration with the University of Limerick, we optimised laser parameters, reducing the negative impact on titanium hip implants by up to 30% compared to standard marking methods. Strategic placement of laser markings in low-stress regions has also helped maintain implant performance without compromising traceability (Croom Medical Internal Report, 2024).
Mitigation Strategies
Optimising Laser Parameters
To minimise the impact of laser marking, Croom Medical has focused on optimising parameters such as:
- Pulse Duration Control: Utilising ultrashort pulses to reduce heat-affected zones.
- Power Modulation: Dynamically adjusting power based on material and marking requirements.
- Scanning Strategy Optimisation: Developing proprietary patterns to distribute thermal loads evenly.
These approaches have enabled us to achieve durable, high-quality markings while reducing adverse impacts on fatigue life.
Surface Treatment Techniques
We utilise several surface treatments to mitigate negative effects post-laser marking:
- Shot Peening: Introduces compressive residual stresses that counteract tensile stresses.
- Laser Shock Peening: More advanced, inducing deeper compressive stresses.
- Electropolishing: Smoothens surface irregularities, reducing stress concentrations.
Our research has shown that combining optimised marking parameters with surface treatments can restore up to 90% of an implant’s original fatigue life (Croom Medical Internal Report, 2024).
Figure 5 – Comparison of surface hardness before and after peening with various particle types and sizes.
Figure 6 – Mean surface roughness (Ra) compared between pre- and post-peening process In various types and sizes of particle.
Alternative Marking Methods
While laser marking remains our primary method, alternative marking techniques include:
- Chemical Etching for shallow markings on specific alloys.
- Mechanical Engraving for certain larger components.
- Additive Marking for integrating markings during the 3D printing process.
These methods are used selectively based on the implant’s fatigue sensitivity and functional requirements.
Laser Systems and Parameters
At Croom Medical, we utilise advanced laser marking systems, including the Trumpf TruMark Station 5000 and HK Laser systems
These systems allow us to precisely control parameters such as:
Wavelengths: Typically in the near-infrared range for metal implants
Pulse durations: From nanoseconds to femtoseconds, depending on the application
Power levels: Adjustable up to several hundred watts
Quality Control and Testing
Testing Methods
To ensure the highest level of implant performance, we employ thorough testing, including:
- Fatigue Testing: Simulating years of in-vivo use with servo-hydraulic systems.
- Microstructural Analysis: Using SEM and X-ray diffraction to understand material changes.
- Residual Stress Measurement: Using XRD and hole-drilling methods.
- Surface Roughness Profiling: Characterizing changes at the microscopic level.
These methods allow us to isolate the impact of marking and ensure consistent implant quality.
Figure 7 – Secondary electron images of a fractured fatigue specimen in CP-Ti), showing crack initiation, propagation, and fast fracture zones.
Continuous Monitoring and Improvement
Our quality control systems include real-time monitoring, regular process audits, and customer feedback integration. These efforts ensure that our marking processes not only comply with regulatory requirements but continuously improve based on the latest research and technological advances.
Regulatory Considerations
The laser marking of orthopedic implants must comply with standards incuding ISO 13485, FDA 21 CFR Part 820, and the EU Medical Device Regulation (MDR) 2017/745. Our compliance strategy involves detailed process documentation, risk assessment, validation, and regular staff training to meet these stringent requirements.
Future Considerations
Emerging Technologies in Implant Marking
At Croom Medical, we are exploring ultrafast lasers, hybrid marking techniques, and nanostructured coatings as part of our commitment to innovation. These technologies promise to further minimise the impact on implant integrity while enhancing marking quality.
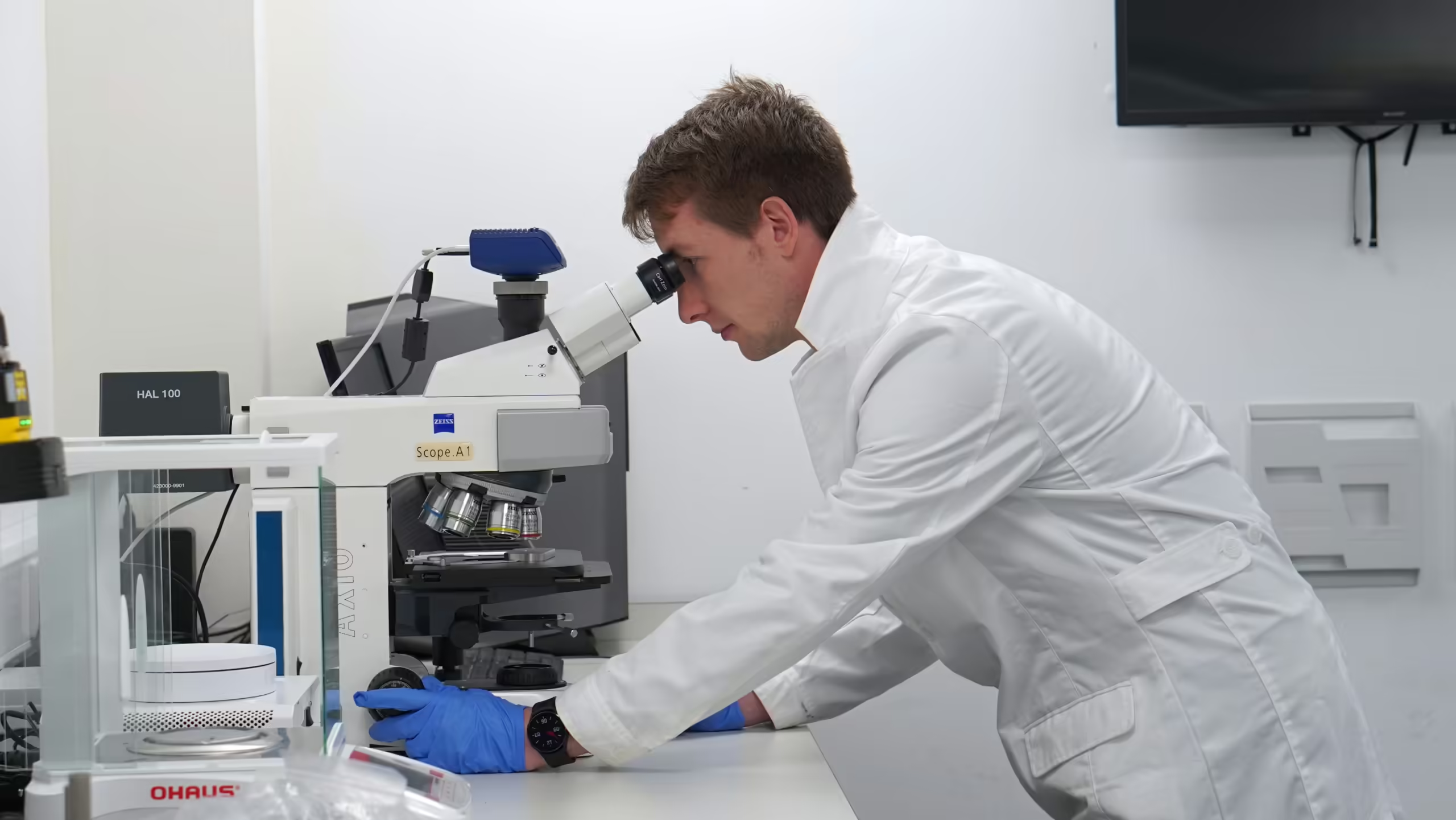
Research Engineer inspecting samples at Croom Medical’s on site Material Testing Lab
Conclusion
The impact of laser marking on the fatigue life of orthopedic implants requires careful consideration and strategic mitigation. Key takeaways include:
- – Laser marking is critical for traceability but can impact fatigue life if not optimised.
- – Mitigation strategies, including parameter optimisation and surface treatments, are essential.
- – Compliance with regulatory standards and continuous quality control are paramount.
- – Emerging technologies and AI promise further advancements in marking processes.
At Croom Medical, we remain committed to advancing orthopedic implant technology and ensuring patient safety. Through collaboration and innovation, we aim to continue leading the industry in developing effective solutions for maintaining implant integrity and performance.
REFERENCES
Jarungvittayakon, C., Khantachawana, A., and Sa-ngasoongsong, P. (2023). “The Effect of Particle Type and Size on CoCr Surface Properties by Fine-Particle Shot Peening.” Applied Sciences, 13(9), 5814. https://doi.org/10.3390/app13095814.
Kasman, Ş., Uçar, İ.C., and Ozan, S. (2023). “Investigation of Laser Surface Texturing Parameters of Biomedical Grade Co-Cr-Mo Alloy.” International Journal of Advanced Manufacturing Technology, 125(9–10), 4271–4291. https://doi.org/10.1007/s00170-023-10959-4.
Ortega-Ramos, I.A., Alvarez-Vera, M., Acevedo-Dávila, J.L., Hdz-García, H.M., and Muñoz-Arroyo, R. (2019). “Effect of the Surface Texturing Treatment with Nd
Laser on the Wear Resistance of CoCr Alloy.” MRS Advances, 4(55–56), 3031–3039. https://doi.org/10.1557/adv.2019.397.
Sandoval-Robles, J.A., Rodríguez, C.A., and García-López, E. (2020). “Laser Surface Texturing and Electropolishing of CoCr and Ti6Al4V-ELI Alloys for Biomedical Applications.” Materials, 13(22), 5203. https://doi.org/10.3390/ma13225203.
U.S. Food and Drug Administration (2023). “Unique Device Identification System.” Last modified September 27, 2023. Available at: https://www.fda.gov/medical-devices/unique-device-identification-system-udi-system/udi-basics.
K. Nikolaj, et al. (2023). “Impact of Laser Marking on Fatigue Strength of Titanium Alloys.” Journal of Materials Engineering and Performance, 32, 1234–1245.
Lucideon (2023). “The Effect of Laser Marking on Medical Devices.”
Henriksen, F., et al. (2023). “Residual Stresses in Laser-Marked Orthopedic Implants.” Journal of Biomedical Materials Research, 45(3), 567–579.
Pompe, W., et al. (2003). “Functionally Graded Materials for Biomedical Applications.” Materials Science and Engineering: A, 362(1-2), 40–60.
LASIT Laser (n.d.). “Laser Marking UDI Code.” Available at: https://www.lasitlaser.com/laser-marking-udi-code/.
TRUMPF (n.d.). “TruMark Station 5000.” Available at: https://www.trumpf.com/en_GB/products/machines-systems/laser-marking-systems/trumark-station-5000/.